Recommendation to perform right heart catheterization in pulmonary hypertension
Joint document by the Federación Argentina de Cardiología and the Sociedad Argentina de Cardiología
Executive committee
Maria Lorena Coronel (FAC)1; Mirta Diez (SAC)2; Luis Roberto Lema (FAC)3; Adrián José Lescano (SAC)4.
Writing committee
Maria Cecilia Moris (FAC)5; Guillermina Sorasio (SAC)6; Diego Federico Echazarreta (FAC)7; Norberto Oscar Vulcano (SAC)8; Arturo Fernández Murga (FAC)9; Nicolás Alejandro Caruso (SAC)10; Daniela García Brasca (FAC)11; Andrés Nicolás Atamañuk (SAC)12; Fernando Daghero (FAC)13; Alejandro Peirone (SAC)14; Lilia Luz del Valle Lobo Márquez (FAC)15; Maria Lujan Talavera (SAC)2; Guillermo Pacheco (FAC)16; Pablo Spaletra (SAC)17
Review committee
Liliana Ethel Favaloro (SAC)18; Sergio Víctor Perrone (FAC)19; Jorge Thierer (SAC)20; Eduardo Roque Perna (FAC)1
1 Heart Failure and Pulmonary Hypertension Division, Instituto de Cardiología J F Cabral, Corrientes. MTFAC
2 Heart Failure and Transplant Section, Instituto Cardiovascular de Buenos Aires, Buenos Aires. MTSAC
3 Pulmonary Hypertension Department, Instituto Modelo de Cardiología Privado SRL, Córdoba. MTFAC
4 Cardiology Department, Sanatorio Trinidad Quilmes. Heart Failure and Pulmonary Hypertension, Centro Gallego, Buenos Aires. MTSAC
5 Heart Failure and Pulmonary Hypertension Service, CIAT. Sanatorio Sarmiento, Tucumán. MTFAC
6 Heart Failure and Pulmonary Hypertension Service, Hospital Rivadavia. Sanatorio Trinidad Ramos Mejía, Buenos Aires. MTSAC
7 Cardiovascular Area. Centro Médico Capital, Buenos Aires. MTFAC
8 Cardiology Service. Hospital Italiano de Buenos Aires, Buenos Aires. MTSAC
9 Hemodynamics Service. Instituto de Cardiología, Tucumán. MTFAC
10 Heart Failure, Pulmonary Hypertension and Heart Transplant Department. Coronary Unit. Sanatorio Trinidad Mitre, Buenos Aires. MTSAC
11 Cardiology Service. Hospital Italiano de Córdoba, FCM-UNC, Córdoba. MTFAC
12 Cardiology Division. Hospital General de Agudos Juan Antonio Fernández. Hospital Universitario Austral, Buenos Aires. MTSAC
13 Pulmonary Hypertension and Pediatric Cardiology Unit. Cardiology Service. Sanatorio Allende, Córdoba. MTFAC
14 Cardiology Department. Hospital de Niños de la Santísima Trinidad. Hospital Privado Universitario, Córdoba. MTSAC
15 Heart Failure and Pulmonary Hypertension Department. Instituto de Cardiología, Tucumán. MTFAC
16 Pulmonary Hypertension and Interventional Cardiology Unit. Sanatorio Allende, Córdoba. MTFAC
17 Interventional Cardiology Service. Instituto Cardiovascular de Buenos Aires, Buenos Aires. MTSAC
18 Heart Failure, Pulmonary Hypertension and Intrathoracic Transplantation. Hospital Universitario Fundación Favaloro, Buenos Aires. MTSAC
19 Instituto FLENI. Instituto Argentino de Diagnóstico y Tratamiento. Hospital El Cruce de Florencio Varela, Buenos Aires. MTFAC, IHF-HFSA
20 Heart Failure Unit, CEMIC. Ciudad Autónoma de Buenos Aires. MTSAC
ABBREVIATIONS
6MWT 6-minute walk test
AVT acute vasoreactivity test
CaO2 arterial oxygen content
CHD congenital heart disease
CI cardiac index
CO cardiac output
CvO2 central venous oxygen saturation
dPAP diastolic pulmonary artery pressure
DPG diastolic pulmonary gradient
dSBP diastolic systemic blood pressure
HR heart rate
LA left atrium
LC lung compliance
LV left ventricle
LVEDP left ventricular end diastolic pressure
mPAP mean pulmonary artery pressure
mSBP mean systemic blood pressure
MV minute volume
PA pulmonary artery
PAH pulmonary arterial hypertension
PAOP pulmonary artery occlusion pressure
PAP pulmonary artery pressure
PAPi pulmonary artery pulsatility index
PVR pulmonary vascular resistance
PVRi pulmonary vascular resistance index
Qp/Qs pulmonary flow/systemic flow
RA right atrium
RAP right atrial pressure
RHC right heart catheterization
RV right ventricle
SaO2 arterial oxygen saturation
SAP systemic arterial pressure
sPAP systolic pulmonary artery pressure
sSBP systolic systemic blood pressure
SV stroke volume
SVI stroke volume index
SvO2 mixed venous oxygen saturation
SVR systemic vascular resistance
TPG transpulmonary pressure gradient
VO2 oxygen consumption
WP wedge pressure
WU Wood units
.
TABLE OF CONTENTS
1. Introduction
2. Definition and hemodynamic classification of pulmonary hypertension
a. Hemodynamic definition of pulmonary hypertension
b. Exercise-induced pulmonary hypertension
c. Hemodynamic classification of pulmonary hypertension
3. Role of right heart catheterization for the diagnosis and monitoring of pulmonary hypertension
a. Cardiac catheterization for diagnosis in patients with suspicion of pulmonary hypertension
b. Cardiac catheterization in monitoring pulmonary hypertension patients
4. Preparation of patients, technique and potential complications of the procedure
a. Site of performance
b. Staff required
c. Equipment
d. Preparation of patients
e. Procedure
f. Puncture guided by echography
g. Venous accesses
h. Complications
i. Aspects to be highlighted
5. Measurement of pressures and determination of cardiac output. Hemodynamic variables
6. Acute vasoreactivity test and fluid overload testing
a. Acute vasoreactivity test
b. Fluid overload testing
7. Hemodynamic assessment in pulmonary hypertension associated to congenital heart disease
a. Eisenmenger syndrome
b. Pulmonary hypertension associated to left-to-right shunt
c. Pulmonary hypertension with small/matching congenital heart defect
d. Postoperative pulmonary hypertension
8. Pulmonary angiography in chronic thromboembolic pulmonary hypertension
1.INTRODUCTION
Right heart catheterization (RHC) is the gold standard to evaluate cardiopulmonary hemodynamics and it is the mandatory procedure to confirm the diagnosis of pulmonary hypertension (PH). Furthermore, it assesses the severity of the disease and its prognosis, enabling a proper risk stratification, and in patients with the indication, it allows to perform the acute vasoreactivity test (AVT), fluid overload testing and pulmonary angiography.
The current definition of PH is strictly hemodynamic. For a proper diagnosis of pulmonary arterial hypertension (PAH), there are three defined variables evaluated invasively by RHC performed in resting conditions: mean pulmonary artery pressure (mPAP) >20 mmHg; pulmonary artery occlusion pressure (PAOP), also known as pulmonary capillary pressure (Pcap) or pulmonary capillary wedge pressure (PCWP); ≤15 mmHg and pulmonary vascular resistance (PVR) ≥3 Wood units (WU)[1,2].
.
It is important to underscore that the interpretation of cardiopulmonary hemodynamics should be considered taking into account the clinical symptoms and the findings from noninvasive, imaging, and biochemical tests, and all others that assess exercise capacity.
RHC is a challenging, complex procedure requiring experience and a thorough analysis of data. To obtain accurate and reproducible information, and to minimize risks, it should be conducted in specialized centers and by operators with a proper education.
The aim of this document made by the Federación Argentina de Cardiología and the Sociedad Argentina de Cardiología about the role of RHC in PH is to provide practical recommendations for a proper performance and interpretation of this procedure. It is addressed at physicians specialized in cardiology, pneumonology, rheumatology, intensive care, infectious diseases, hematology, hepatology, cardiovascular surgeons and interventional physicians. Its implementation will contribute to perform an efficient and safe RHC, which will provide full information, thus helping to an optimal management of PH.
2. DEFINITION AND HEMODYNAMIC CLASSIFICATION OF PULMONARY HYPERTENSION
RHC allows to make a confirming diagnosis of PH, to differentiate precapillary compromise from postcapillary compromise, to evaluate hemodynamic severity and to perform, when indicated, AVT and/or fluid overload testing and/or pulmonary angiography[1].
2.1 Hemodynamic definition of pulmonary hypertension
In the first World Symposium on Pulmonary Hypertension in Genoa in 1973, PH was defined arbitrarily and by expert consensus as a pathological condition with an mPAP ≥25 mmHg, measured by RHC, in supine position and resting[3].
.
This definition was maintained over the years, and it was recommended in the different guidelines and clinical trials. In the most recent World Symposium on PH held in Nice in year 2018, it was proposed to reduce the cutoff point for the diagnosis of PH to mPAP >20 mmHg[2]. One of the arguments for this recommendation was based on the studies by Kovacs et al, who in a systematic review of 47 studies, determined that normal mPAP in healthy individuals is 14±3.3 mmHg, regardless of race or ethnicity. Thus, 2 standard deviations above this value in a normal distribution suggests that mPAP of 20 mmHg is the upper limit for the normal value (above percentile 97.5)[4].
About this point, it is important to highlight that mPAP in isolation cannot characterize a clinical condition and does not define a pathological process per se, as it could be altered by other conditions, as increase in cardiac output (CO); in PAOP or wedge pressure (WP) and by left-to-right shunts. In this scenario, since the third World Symposium on PH, it is advised to add PVR to the hemodynamic definition, to properly identify precapillary PH, suggesting pulmonary vascular disease[5].
All in all, and according to the proposal by the last symposium on PH, the definition of PAH includes mPAP >20 mmHg, with PVR ≥3 WU, maintaining the same value used traditionally for PAOP (≤15 mmHg), thus defining precapillary PH, including different groups in the clinical classification (groups 1, 3, 4 and 5)[2]. Adding PVR to the definition of PAH is extremely relevant when differentiating between increase in pulmonary pressure values by hyperflow phenomenon, pulmonary vascular disease with compromise of arterial vascular remodeling, and isolated postcapillary component (PH groups 2 and 5)[1,2]. Differentiating these entities is essential, as incorporating the specific treatment for PH has been favorable for PAH and has shown no benefits in postcapillary PH (Table 1). Finally, the new definition of PAH would enable to identify pulmonary vascular disease earlier, and therefore, to define a quick diagnostic and therapeutic strategy in selected patients.
2.2 Exercise-induced pulmonary hypertension
Different studies suggest that mPAP normally does not exceed 30 mmHg in the presence of cardiac output of less than 10 L/min during exercise[6]. However, it has not yet been possible to reintroduce a clear definition of exercise-induced PH. The challenge of obtaining accurate measurements with exercise during RHC (CO, PAOP) and the lack of diagnostic discrimination power about whether it is due to elevated PAOP (PH group 2) or pulmonary vascular disease, make the definition of exercise-induced PH definition difficult as an entity. A proposal of definition, according to the last World Symposium of PH is: mPAP ≥30 mmHg and total PVR ≥3WU.
2.3 Hemodynamic classification of pulmonary hypertension
Discriminating the different hemodynamic subtypes is a priority, as pathophysiology, clinical presentation and treatment are different. The hemodynamic classification of PH is presented in Table 1.
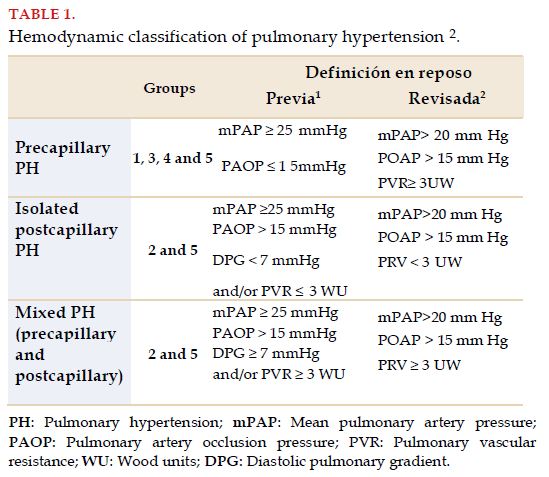
Precapillary PH includes the clinical groups of PAH (Group 1), PH due to chronic pulmonary disease or hypoxia (Group 3), chronic thromboembolic pulmonary hypertension (CTEPH) and other pulmonary artery obstructions (Group 4) and multifactorial PH (Group 5).
Postcapillary PH includes PH due to left heart disease, which could be isolated or mixed and PH by multifactorial causes.
3. ROLE OF RIGHT HEART CATHETERIZATION FOR THE DIAGNOSIS AND MONITORING OF PULMONARY HYPERTENSION
3.1 Cardiac catheterization for diagnosis in patients with suspicion of pulmonary hypertension
The measurements of all minor circulatory circuit pressures were, since 1987, the gold standard for the diagnostic certification of PAH. It is proposed to carry out this procedure when there are sound suspicions of the disease and there is a clinical context according to the support of compatible noninvasive tests, mainly echocardiographic. The echocardiographic probability of PH (low, intermediate or high) and the presence of risk factors for PAH or CTEPH lead management toward the performance of RHC (Table 2)[1,7].
During RHC, it is possible to carry out provocative maneuvers to better characterize the disease, confirm the diagnosis, assess severity, approach the etiology and guide management[1]. When this procedure is made in experienced centers it has an extremely low morbimortality[8].
The measurements that should be taken are right atrial pressure (RAP), right ventricle (RV), systolic pulmonary artery pressure (sPAP), diastolic pulmonary artery pressure (dPAP), mPAP and PAOP, as well as CO.
It is essential for a better characterization of PH and PAH, to record the hemodynamic variables drawn from cardiac index (CI), transpulmonary pressure gradient (TPG), diastolic pulmonary gradient (DPG), systemic vascular resistance (SVR) and PVR.
Mixed venous oxygen saturation (SvO2) should be obtained in the superior and inferior vena cava and the pulmonary artery (mixed venous blood). If there is a suspicion of shunt, it is useful to measure oxygen saturation in the right atrium (RA) and the RV and in the left chambers and the aorta. In such cases, measuring CO by Fick’s method is more reliable than thermodilution[9,10,11,12,13].
It is recommended to associate RHC with coronary angiography when there are clear cardiovascular risk factors or evidence of CAD, echocardiographic signs of systolic and/or diastolic left ventricular dysfunction. On the other hand, left heart catheterization, along with the quantification of left ventricular end diastolic pressure (LVEDP), are indicated when the PAOP found does not match clinical data or cannot be quantified[10].
Performing RHC requires a precise technique and should be carried out by experienced staff. The zero hemodynamic point of reference should be taken at the end of non-forced expiration, in supine position, in the thoracic mid-line, halfway between the sternum and the bed surface[9,10,11].
It is convenient for the values found to be shown in graphs with curves, since such morphology provides diagnostic information and it is convenient to correlate them with additional data, essentially echocardiographic to prevent mistakes. For instance, right ventricular failure may magnify the RA curve, since this cardiac chamber should contract against an elevated RV end-diastolic pressure; likewise, a prominent “v” wave indicates the existence of tricuspid valve regurgitation[11].
It is advisable to perform balloon inflation of the Swan Ganz catheter at the RA level, and advance until the wedge point. The certainty of a proper location is provided by a blood sample taken at this point, the saturation of which should be equal to that of the left atrium; i.e., equal to the systemic one. An average of three successive measurements is considered valid in patients with sinus rhythm and up to five of them with atrial fibrillation[12].
The percentage of its diagnosis or incorrect classification increases when the procedure protocol is not followed thoroughly, increasing the risk of a wrong treatment[14,15].
When PAH is idiopathic, hereditary or induced by drugs or toxins, RHC should be accompanied by AVT to indicate a treatment with calcium channel blockers in high doses, in the case this test is positive. When the cause of PAH is due to another etiology, these drugs do not have a favorable effect in the long term or may even be harmful[1,16].
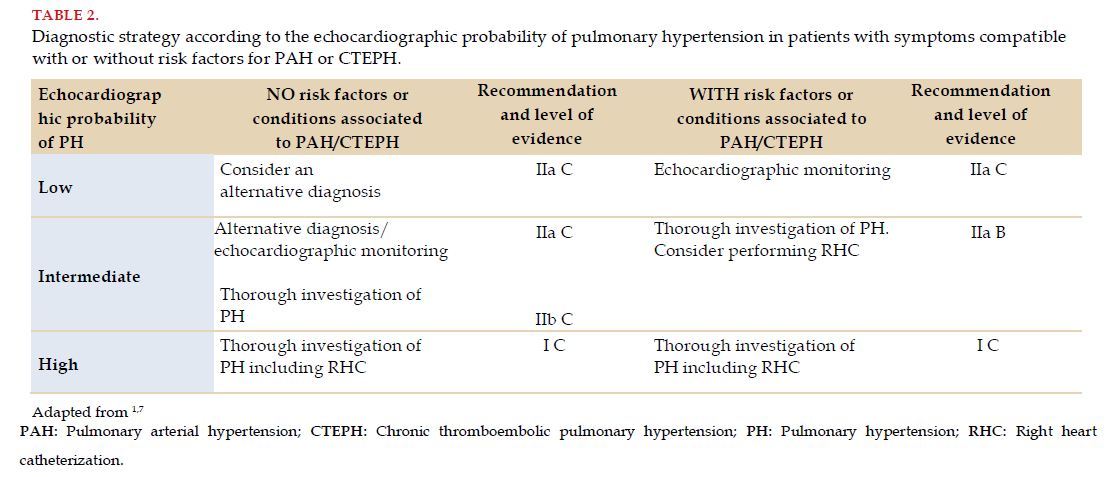
3.2 Cardiac catheterization in the monitoring of patients with pulmonary hypertension
A comprehensive approach of PAH to stratify individual risk periodically includes assessing the following variables: the presence of heart failure, velocity of symptom progression, syncope, functional class, distance walked in the 6-minute walk test (or cardiopulmonary exercise test), serum values of NT-proBNP/BNP, right chamber remodeling, the presence of pericardial effusion, and finally, the behavior of several hemodynamic variables (RAP, CI and SvO2)[1,17].
Other factors with impact on the symptoms and prognosis of the disease that are not affected by the specific therapy established in PAH are age, gender, underlying disease and comorbidities. In spite of individual predictions being difficult, patients are classified as in low, intermediate and high risk according to the estimated mortality in one year being less than 5%, between 5-10% and more than 10%, respectively[1,18].
The variables that accompany evolution may not behave consistently, and positions the patient in different risk categories. Once again, it is individual evaluation that should drive personalized therapeutic decisions[19].
In this scenario, hemodynamic variables play a fundamental role at the time of follow-up, defining a given risk factor[20,21,22].
The current guidelines and consensuses recommend performing RHC as the necessary method for the diagnosis of PAH and as part of baseline evaluation. About follow-up, it is advised to do it every 6 or 12 months in conditions of stability, or every 3 to 6 months after clinical worsening or modification in therapy (Table 3)[1,7].
Baseline hemodynamic variables do not predict the risk of death or transplant in a scenario of PAH. There are three hemodynamic parameters of RHC that contribute prognostic value: RAP, CI and SvO2[1,23]. After the initial treatment, the stroke volume index was reported as an independent prognostic variable and has proven to have more predictive value than CI or pulmonary artery distensibility, even when CI is within the range of low risk (≥2.5 L/min-1/m-2)[22]. Thus, hemodynamic variables remain essential for a multidimensional evaluation of the prognosis and response to the treatment of this pathology.
Some of the mentioned procedures present a high complexity and require experience to perform them, so there is consensus that patients with PAH should be referred to reference units to undergo these measurements and for them not to suffer unnecessary repetitions or avoidable complications[8,14].
There is wide experience drawn from numerous studies that included a considerable number of patients with PAH, supporting the prognostic significance of hemodynamic variables in comparison to the relatively small number of studies where biomarkers were used, as well as magnetic resonance and echocardiographic measurements for risk evaluation, associated to a lack of homogeneity in the noninvasive parameters analyzed. Therefore, the usefulness of RHC for routine follow-up is supported by the magnitude and weight of the evidence available currently[22,23,24,25]. Although it is not indicated to be applied as a routine during follow-up for a small proportion of patients meeting the noninvasive criteria of low risk, it provides important information for treatment choices in patients in higher risk in prognostic scales[23].
4. PREPARATION OF PATIENTS, TECHNIQUE AND POTENTIAL COMPLICATIONS OF THE PROCEDURE
Before the performance of RHC for diagnosis or monitoring in PH, it is indispensable to thoroughly know the clinical history and auxiliary tests, particularly when taking into account that the results and conclusions of this study usually entail important therapeutic and prognostic decisions.
For conventional vein punctures, suspension of anticoagulation is not necessarily indicated. However, it is suggested to perform vascular Doppler ultrasound-guided puncture in such cases, to reduce the risk of complications. In the case of considering the performance of associated arterial catheterization, suspending anticoagulation is recommended according to the established protocols. Both the size and the pressure in central venous accesses are very influenced by the level of blood volume. Therefore, it is suggested to carefully assess each case to define the indication of diuretics and fluid ingestion before the procedure[12,26,27].
4.1 Site of performance
RHC should be made in third-level centers, where there are multidisciplinary services for the management of these patients, with specific areas and monitoring equipment to take action fast in the case of complications (hemodynamic rooms, intensive care units with availability of all current technology). Although it is true that this procedure can be conducted in an intensive care unit, it is important for the first catheterization to be performed in a hemodynamic laboratory, where the possibility is there to implement all the technical variants required for a proper diagnosis; for instance, taking oximetric samples guided by radioscopy, performing right/left heart catheterization and arteriography.
4.2 Required staff
It is important for RHC to be performed by a multidisciplinary team (cardiologist, pneumonologist, interventional cardiologist) with a deep knowledge on the pathology, with experience in performance and PH management, as these are risk patient who do not tolerate complications well[8].
4.3 Equipment
To generate a sterile environment, antiseptic (chlorhexidine, povidone-iodine) applicators are required, a fenestrated sterile field, surgical mask, cap, safety goggles, scrubs and sterile gloves.
To proceed to place the introducer sheath, the following are needed: swabs, sterile saline solution, lidocaine, syringes of 5 or 10 cm3, 25G (0.5 mm) needles, 18G introducer needle or 16G or 18G Abbocath, guidewire, a scalpel, valved introducer sheath with its dilator, suture, scissors and adhesive tape.
Since using ultrasound is advisable to facilitate locating the central vein, a sterile cover and conductive gel will be required during puncturing.
To place the pulmonary artery catheter (Swan Ganz), the following are required: catheter, cuff, sterile saline solution, infusion ports and proper three-way stopcock valves, invasive blood pressure transducer and electronic monitor, preferably capable of recording multiple measurements simultaneously (invasive and noninvasive blood pressures, oxygen saturation, long-term ECG recording) and several heparinized syringes to take blood samples for oximetry.
The pulmonary artery catheter measures 110 cm of length, with a diameter that may range between 5 and 8 French, according to characteristics and design. All models have a distal port (usually yellow) connected at the tip. Furthermore, most present a proximal port (in general blue) connected to a lumen at a distance of 30 cm from the tip of the catheter. Others may have accessory ports to perform infusions that also usually have their lumens at 30 cm from the tip.
The balloon-tipped catheter is inflated when injecting air through the port to do this (usually red). It is advised to use the specific syringe provided, since it can inflate only the balloon capacity. To reduce the mechanical trauma during placement, the inflated balloon should completely surround the catheter tip. Be sure that the balloon is completely inflated when advancing with the catheter, and completely deflated when drawing back.
Since the distal end may induce ventricular arrhythmias, there should always be a defibrillator and intravenous temporary pacemaker.
If fluoroscopy is used, each operator or assistant should use a lead apron and a thyroid shield[9,27,28,29,30,31].
4.4 Preparation of patients
It is important to inform patients about the characteristics of the test, of the significance it has for the diagnosis and treatment of their disease, of the discomforts and possible complications that may appear through it. Next, the informed consent signature is required. Subsequently, review the control list to confirm the personal information and clinical condition, the procedure to perform and availability of all the required material and equipment.
Place the patient in supine position and choose the most appropriate access. Right internal jugular vein access or subclavian vein or left brachial accesses are preferable, since the catheter curvature facilitates its passage from the insertion until the pulmonary artery.
Wash hands with antimicrobial solution and wear the scrub and the gloves. Sterilize the work area with chlorhexidine and povidone iodine and place the sterile field. In the case of requiring an ultrasound probe to locate the vein to puncture, place the probe within the sterile cover. Clean the introducer and the pulmonary artery catheter with saline solution, inflate the balloon and introduce it in a container with saline solution to confirm the absence of leaks.
4.5 Procedure
Use the 25G needle to infiltrate skin and subcutaneous tissue with lidocaine. Advance with the 18G needle or Abbocath toward the vein, while negative pressure is applied on the syringe. In the case of using ultrasound, you may directly visualize the needle entering the vein. When aspiring dark red blood, non-pulsatile blood, remove the syringe (and the needle in case of using Abbocath) and insert the wire through the needle or the Abbocath (Seldinger technique). Use the scalpel (preferably with number 11 blade) to penetrate the skin adjacent to the needle, parallel to it, and remove the needle. While the wire is being held to guarantee the access and prevent embolization, insert the introducer sheath together with the dilator through the wire until it holds the space of the wound. Remove the guide and the dilator of the introducer and wash with saline solution to ensure a proper flow[27,28,28,30,31].
In the case of not having an ultrasound machine to locate the vein, it is possible to perform a search for it with the 25G needle after having injected the anesthetic and applying negative pressure on the syringe, and once located, use the 18G needle or the Abbocath and continue with the Seldinger technique as described.
There are also Rowlerson syringes that allow guidewire access through the plunger of the syringe, not requiring withdrawing the syringe from the puncture needle (a procedure that sometimes causes it to lose the access achieved).
4.5.1 Ultrasound-guided puncture
It is convenient to use a portable ultrasound machine that enables locating the access vein, detecting possible anatomical variations and assessing permeability.
It has been proven that using these machines significantly reduces the number of complications of the procedure, such as the accidental puncture of the carotid artery[32].
The ultrasound-guided catheterization method could be divided into different steps[33].
- Mapping or scanning the chosen region to make the puncture: to locate the vein, evaluate diameter and depth and ensure permeability.
- Although veins may present systolic expansion due to the passive transmission of an adjacent artery or in cases of significant tricuspid valve regurgitation, its collapsible character with extrinsic compression differentiate them from arteries.
- Using ultrasound gel and sterile cover to wrap around the transducer to ensure the sterility of the puncture.
- Advance slowly with the puncture needle or Abbocath to visualize its trajectory and direct it toward the vein lumen. Applying negative pressure with the syringe, once the needle is within the lumen, it will fill with dark red blood guaranteeing venous access.
- Advance with the wire following the Seldinger technique and guarantee by ultrasound that the wire is within the vein, both in the transversal and the longitudinal views.
- Continue with the procedure, as described above.
4.5.2 Venous accesses
The venous accesses usually used are the right jugular, the right femoral and the left brachial or subclavian. Although jugular access is the one most frequently described in literature and the one most widely used, the choice of access will depend on the availability of imaging methods (ultrasound, radioscopy), individual characteristics of each patient, and particularly, the experience and familiarity of the operator with each access (Table 4).
4.6 Complications
When performed in experienced centers and with a history in the management of PH, RHC is associated to very low rates of complications and death. In this regard, several registries at world level report a rate of severe complications close to 1%, with a mortality close to 1/10,000 catheterizations made[8].
In fact, most complications described are usually mild to moderate, more commonly associated to those derived from venous access, vagal reactions or effects secondary to the drugs used in AVT[8,26].
The most common initial complications include ventricular arrhythmias and complete right bundle branch block, which are usually self-limited. Complete atrioventricular block may occur in patients with previous complete left bundle branch block. Exceptionally, embolization may occur of the wire used for the Seldinger technique and become inaccessible, or the catheter may tangle in a cardiac chamber and prevent its withdrawal. In any of these cases, the vascular surgeon should be consulted to ensure a safe withdrawal.
Air embolism may occur if the catheter ports were not properly washed with saline solution or if the previously washed catheter or introducer are kept open. Patients may present dyspnea, precordial pain, tachycardia, hypotension and even increase in right chamber pressures. For such situations, patients should be put in a Trendelenburg position to limit air release from the right ventricle and supplemental high flow oxygen should be administered to promote air reabsorption. In more severe cases, it may be necessary to use hyperbaric oxygen therapy.
Pulmonary artery rupture is a very rare condition, but potentially fatal. The incidence reported may reach 1 in 3,000 catheterizations performed. The risk factors to present it include: advanced age, prolonged balloon inflation, PH and anticoagulation requirements. Patients may present hemoptysis, severe hypoxemia and shock. When no immediate actions are taken, there is a high risk of death. The balloon should be maintained inflated to limit major bleeding. Intubation, preferably with double-lumen endotracheal tube, and position it in lateral decubitus, with hemithorax affected downwards. Consult urgently with the chest surgeon.
Finally, later the following may be verified: pulmonary infarction, infections related to the catheter and thrombosis. To reduce the risks of pulmonary infarction, be sure that the catheter tip is located in such a way that complete balloon inflation is required to measure PAOP, and be sure to deflate it after PAOP measurement is over[8,26,31,34].
4.7 Aspects to highlight
Taking into account that performing RHC is a fundamental tool to define managements, it is particularly important to know thoroughly the technical issues related to the procedure. Proper, systematic and protocolized work, experience in performance of the procedure, and knowledge and management of potential complications are particularly relevant to obtain the best results from the test.
5. MEASUREMENT OF PRESSURES AND DETERMINATION OF CARDIAC OUTPUT. HEMODYNAMIC VARIABLES
To guarantee a proper estimation of hemodynamic parameters measured or estimated from RHC, we should pay particular attention to procedure protocols and perform them in a standardized manner.
RHC should include a full hemodynamic evaluation, including direct measurement of PAP, PAOP, RAP, RV pressure, CO or MV, and SvO2. The parameters estimated from these include TPG, DPG, PVR, SVR, CI, lung compliance (LC), stroke volume (SV), stroke volume index (SVI) and pulmonary artery pulsatility index (PAPi) (Table 6).
As diagnostic criteria are categorically based on specific values, an essential aspect at the time of making measurements is a proper recording of pressure values. For this, pressure transducer position is essential, as there is a great variability in leveling or zeroing procedures. The absence of standardization in regard to the exact position of the transducer may cause differences of up to 8 mmHg in the registries of reported pressures[35].
To unify criteria, it is advised to place the pressure transducer and level to zero in the middle thoracic line, with a reference point defined by the intersection of three transthoracic planes: the frontal plane at the middle thoracic level, transversal plane at the level of the fourth anterior intercostal space and the middle sagittal plane (Figure 1)[11,26]. Therefore, and more than 70 years later, “Burch’s phlebostatic axis” remains valid[36].
The positioning of the Swan Ganz catheter is characterized by its vascular trajectory until its final position and thanks to this, it allows to record, in a logical and systematic manner, pressure values in the different chambers as we advance (RA, RV, PA and PAOP).
Connecting the distal port of the Swan Ganz catheter to the pressure transducer and with the zeroing already done, the catheter advances 15 cm, so the tip is outside the introducer sheath and the balloon is inflated. It continues to advance until the transduction of a right atrial wave, at a distance of 15-20 cm from jugular access, or 40-50 cm from femoral access. It is important to emphasize that the catheter can be positioned by two techniques; radioscopy or identification of pressure waves in the monitor. The former is easier and faster, and required from the infrastructure and use of X-rays. The latter does not require further tools, other than a monitor to record pressure curves, but it is more difficult, and requires additional expertise to identify the curves according to the cardiac chamber the catheter is going through.
The RA pressure curve has several identifiable components: the “a” wave (generated by atrial contraction or systole), the “c” wave (caused by the bulging of tricuspid valves toward the RA and is linked to the isovolumetric contraction), the “x” descent (due to the descent of the tricuspid plane and linked to ventricular ejection), the “v” wave (produced by the gradual filling of the atrial chamber through venous return) and finally, the “y” descent (indicating RA emptying thanks to the rapid passive RV filling) (Figure 2).
Next, the catheter advances another 5 cm, until the appearance of the RV wave with a change in the recorded pressure value and increase in width and shape of the curves visualized in the monitor (an ascending slope is observed, first slow and then fast, and rapidly descending, representing RV contraction and relaxation) (Figure 3).
Finally, the catheter advances 5 to 10 cm more, until reaching the PA; this waveform presents a rapid ascending movement and a slow descending ramp, with the addition of the dicrotic notch, representing the closure of the pulmonary valve (Figure 4)[37].
To measure PAOP, term preferred to pulmonary capillary pressure, it is advised to inflate the balloon in the RA, from where the catheter is advanced until it reaches the PAOP position (repetitive inflation and deflation in the wedge position should be avoided because of the risk of pulmonary artery rupture)[38]. PAOP should be recorded subsequently as the mean of the measurements at the end of expiration. The recommendation at the end of expiration is due to the pressures in the intra- and extra-thoracic airway being equal at the time. At the end of expiration, thoracic volume corresponds to the residual functional capacity (sum of residual volume and expiratory reserve volume) and there is a minimal influence of the lung elastic recoil in pulmonary pressure readings when functional residual capacity is reached[26]. We must take into account that the PAOP curve presents a recording of waveform similar to that of the RA, as it expresses the LA pressure; therefore, the value to take into account is the one obtained at the end of expiration, and also the end of the “a” wave should be recorded.
There are some factors that should be taken into account when conducting direct measurements of cardiopulmonary hemodynamics, particularly PAOP, since they can lead to an inexact value, resulting in a mistaken classification of the patient with PH (Table 5).
With the catheter end in the PA, blood is aspired through the distal port of the catheter to measure SvO2 and then CO is measured.
The two most used techniques to measure CO are thermodilution and Fick’s method. Thermodilution entails injecting a bolus of saline solution, with predetermined volume and temperature, less than those of blood, through the proximal lumen of the catheter (located at the RA level). The bolus is mixed with blood coming from venous return and modifies its temperature by thermal exchange between fluids. The mixed fluids advance thanks to the RV pumping and the thermistor located at the distal end of the flotation catheter in the PA records the temperature of the mixed fluids. The automatic software of CO integrated in the monitor allows to estimate the CO.
However, the first method to estimate cardiac output was described by Fick in 1887, based on the arterial content of oxygen (CaO2), the oxygen content in mixed venous blood (CvO2) and oxygen consumption (VO2) according to the following formula:
Fick’s method to determine CO requires the following measurements:
- Oxygen consumption (VO2), estimated according to gender, age and body surface.
- Although veins may present systolic expansion due to the passive transmission of an adjacent artery or in cases of significant tricuspid valve regurgitation, its collapsible character with extrinsic compression differentiate them from arteries.
- Using ultrasound gel and sterile cover to wrap around the transducer to ensure the sterility of the puncture.
- Advance slowly with the puncture needle or Abbocath to visualize its trajectory and direct it toward the vein lumen. Applying negative pressure with the syringe, once the needle is within the lumen, it will fill with dark red blood guaranteeing venous access.
- Advance with the wire following the Seldinger technique and guarantee by ultrasound that the wire is within the vein, both in the transversal and the longitudinal views.
- Continue with the procedure, as described above.
The formula to determine CO is:
Where, 1.36 is the capacity to transport O2 of hemoglobin (1.36 ml of O2 per gram of hemoglobin); SaO2 corresponds to arterial oxygen saturation and SvO2 is mixed venous saturation; 10 is the conversion factor form dl to liter.
Studies have shown a reasonable correlation between thermodilution and Fick’s method to estimate CO; although there could be a significant variation in individual patients. In states of low output and/or tricuspid valve regurgitation, thermodilution tends to overestimate cardiac output. In such cases and in the presence of intracardiac shunts, Fick’s method is preferred since thermodilution is inexact.
By means of the recorded values of pressure and CO estimations, we may calculate SVR, PVR, CI, SV, SVI, PAPi and LC.
The estimation of resistances is done based on the analogy with Ohm’s law of electric circuits, with a directly proportional relationship to pressure gradient; and inversely proportional to CO (Table 6)[39].
LC is an expression of distensibility of the pulmonary artery and its large branches; although usually the term “compliance” from English is used in Spanish, we should not forget that it should be interpreted as an inverse expression of the dynamic RV afterload. Therefore, a decrease in it implies an increase in systolic work for the RV[40]. There is still no clearly established consensus for the use and/or value of LC[41]. However, its prognostic role has been described along with other estimations such as pulmonary artery pulsatility index (PAPi) and stroke volume index (SVI), which could be added to the traditional hemodynamic variables[22,23,40,41,42,43,44,45,46,47].
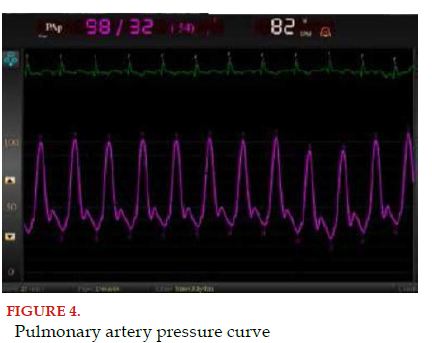
d: Dicrotic notch
6. ACUTE VASOREACTIVITY TEST AND FLUID OVERLOAD TESTING
6.1 Acute vasoreactivity test
In a series of 64 patients published in 1992, Rich et al, reported that patients with a positive vasodilator response to calcium channel blockers presented an exceptionally good survival when treated with these drugs in the long term[48,49].
In 2005, Sitbon et al, showed in a large series of 557 cases with PH, that it is possible to observe an acute vasodilator response in 12.5% of idiopathic PAH, and 6.8% present clinical and hemodynamic improvement in the long term with calcium channel blockers. This study also identified the best criteria to define a positive response: a reduction in mPAP ≥10 mmHg to reach an absolute value ≤40 mmHg, with cardiac output increased or unchanged[50]. These criteria remain valid to this date[1].
AVT should be conducted during RHC and in PH reference centers. It provides prognostic information and it allows to identify patients adequate to receive treatment with high doses of calcium antagonists. It is indicated in PAH when idiopathic, hereditary or induced by drugs/toxins[1,7,51,52].
For pulmonary vascular reactivity tests, nitric oxide inhaled at 10-20 ppm is the preferred agent, but IV epoprostenol, IV adenosine or inhaled iloprost may also be used (Table 7)[7].
A proper response (or positive response) in the long term to calcium channel blockers was defined by improvement in functional class (I or II) and sustained hemodynamic improvement after at least 1 year of treatment. The latter refers to the values found in a second control catheterization that should be equal or better than those obtained in the original acute vasoreactivity test (in general a mean pulmonary arterial pressure <30 mmHg with increased or normal cardiac output)[1,2,50].
The pathophysiology of PAH with positive vasoreactivity is largely unknown. Recently, Hemnes et al, have proven that PAH with positive vasoreactivity and favorable response to calcium channel blockers in the long run is characterized by specific qualities (by studying RNA in peripheral blood lymphocytes in these patients) and different genetic variants in comparison to idiopathic PAH (nonresponders). These results suggest a specific entity with a distinctive clinical course, characterized by a significantly better outcome, better management and different pathophysiology[52,53].
For the reasons mentioned previously (practical tool for diagnosis, better outcome, specific treatment and apparently molecular and genetic bases of its own), the recommendations from the last Nice symposium from 2018 propose to classify them as a different subgroup within group 1 of PAH, called PAH with long-term response to calcium channel blockers[2]. It is specifically in this group where it is justified to continue using this type of drugs as monotherapy[50,54].
The usefulness of AVT and the long-term treatment with calcium channel blockers in PAH associated to other conditions is less clear than idiopathic PAH and is not recommended. In this subgroup of patients (PAH associated to connective tissue disease, HIV, portal hypertension, congenital heart diseases) the results of this test could be misleading and long-term responders are very rare, which would delay the onset of an effective specific therapy[16].
In groups 2, 3, 4 and 5 of the clinical classification of PH it is also not recommended to perform AVT.
Calcium channel blockers that have been used predominantly in the published studies are nifedipine, diltiazem and amlodipine. The daily doses of these drugs that have been efficient in PAH are relatively elevated: 120-240 mg for nifedipine, 240-720 mg for diltiazem and up to 20 mg for amlodipine. It is advisable to start the treatment with a low dose; for instance, 30 mg of an extended-release nifedipine formulation twice a day or 60 mg of diltiazem thrice a day, or 2.5 of amlodipine once a day, to increase it later with precaution and progressively until reaching the maximum dose tolerated. The factors limiting dose increase are usually systemic hypotension and peripheral edema in lower limbs[1].
Patients with PAH that meet the criteria for positive vasodilating response and are treated with calcium channel blockers should be strictly monitored in terms of safety and efficacy, with a first reassessment at 3-6 months of treatment, which will include RHC to confirm the favorable response. In the case of not presenting a proper response, a specific treatment for PAH will be started. Calcium channel blockers should not be used in cases with negative AVT[1,7,50].
6.2 Fluid overload testing
In normal conditions, PAOP is very similar to dPAP, with a mean value of 8.0±2.9 mmHg. So, if we consider 2 standard deviations, a value >15 mmHg is pathological and is defining of postcapillary PH presence[55,56].
Fluid overload testing has the goal of unmasking the presence of postcapillary PH, in a scenario of patients with intermediate/high pre-test probability (Table 8), when PAOP is in an intermediate value in RHC (13.15 mmHg)[10].
In the presence of a heart disease that may generate a reduction in left ventricular compliance (heart failure with preserved ejection fraction, secondary to ischemic heart disease, hypertension, restrictive cardiomyopathy, valve pathology, among others), fluid overload testing will generate a rapid increase in PAOP. It is important to highlight that volume overload may elevate PAOP even in the absence of pathology; the degree of increase depends on multiple variables such as gender, age, the amount of volume administered, infusion velocity, among others[57,58,59,60].
Therefore, it is difficult to standardize the procedure and determine the cutoff values for this test. According to the most relevant publications, it was determined that the test should be conducted with 500 ml of saline solution infused over 5 minutes. And, arbitrarily, a PAOP cutoff value was established >18 mmHg as pathological and indicative of postcapillary PH[10,58,61].
7. HEMODYNAMIC EVALUATION IN PULMONARY HYPERTENSION ASSOCIATED TO CONGENITAL HEART DISEASE
When evaluating a patient with PAH associated to congenital heart disease (CHD), different clinical scenarios and/or anatomical variations could be found. In such context, a standardized RHC (including oximetry in all cardiac chambers) is essential for decision-making, particularly the feasibility of shunt closure.
In CHDs, pulmonary pressures may increase due to several reasons: increase in pulmonary venous pressure (cor triatriatum), increase in pulmonary flow (left-to-right shunts), increase in PVR (Eisenmenger syndrome) or a combination of several or all these factors. The diagnosis of PAH associated to CHD is made just in other types of PAH: mPAP >20 mmHg, PAOP ≤15 mmHg and PVR ≥3 WU and is part of group 1 in the clinical classification of PH[2,62].
In turn, PAH associated to CHD has a classification of its own (Table 9), as well as an anatomo-pathophysiological classification of congenital shunts that enable individualizing the diagnosis in each patient, while enabling a better understanding and categorization of CHD (Table 10). The latter classification emphasizes the type and location of the defect, the size and direction of the shunt, factors to take into account to evaluate these patients[1,63,64].
The development of pulmonary vascular disease is more frequent in large defects, mainly post-tricuspid ones, which allow a leveling of pressures between both ventricles and/or the pulmonary artery. Large defects at atrial level also may cause PH. Although the size of the defect is one of the factors to consider, it is important to differentiate between anatomical and hemodynamic size. Form the anatomical point of view, an interatrial communication is considered small if <2 cm and an interventricular one is <1 cm. These are random values, established by a committee of experts based on clinical experience. For this reason, when evaluating systemic-to-pulmonary artery shunts, the hemodynamic characteristics of the defect are more relevant than anatomical features. A pre-tricuspid shunt is hemodynamically significant when there is dilatation of right chambers in the presence of Qp/Qs (pulmonary blood flow/systemic blood flow) ≥1.5/1. A post-tricuspid shunt is considered relevant at hemodynamic level when there is dilatation in right chambers in the presence of Qp/Qs ≥1.5/1. The defects that are not hemodynamically significant are called restrictive defects. The direction of the shunt is also important when deciding the management, since left-to-right ones may benefit from closing the defect while those from left-to-right will receive pharmacological treatment[1,63.65,70].
In the evaluation by RHC of patients with shunts, the estimation of CO should be made using Fick’s method and not by thermodilution. Fick’s method allows the estimation of CO using different parameters; among them, basal oxygen consumption of the patient[68,69,70]. Likewise, a correct interpretation of RHC results is important to properly estimate the magnitude of the shunt and PVR and indexed PVR (PVR multiplied by body surface area)[9]. Occasionally, the interpretation of hemodynamic results could be complicated, even in expert centers, particularly in patients with moderately high PVR or multiple pulmonary flow sources[71,72]. Also, it is important to perform oximetry in all cardiac chambers and vessels (superior and inferior vena cava, RA, RV, PA, pulmonary veins and systemic arterial). For this reason, whenever possible, it is advised for RHC to be made by a center with experience[8].
7.1 Eisenmenger syndrome
In the presence of post-tricuspid defect, a bidirectional or right-to-left shunt in echocardiogram is diagnostic of Eisenmenger syndrome. However, the same finding in pre-tricuspid shunts may reflect an increase in PVR or increase in telediastolic RV pressure in the presence of restrictive RV, so it is advisable to carry out RHC. From the hemodynamic point of view, Eisenmenger syndrome is defined as PVRi of more than 8 WU/m2 or PVR/SVR (systemic vascular resistance) ratio greater or equal to 1.15[73,74].
7.2 Pulmonary hypertension associated to left-to-right shunt
Deciding who will benefit from closing a defect (surgically/percutaneous) requires experience in the management of these individuals. PH could be secondary to pulmonary hyperflow or elevated PVR. The differentiation between these two scenarios is extremely important since the management will be completely different.
In spite of efforts made in the last decades to look for sound criteria based on evidence to decide on the operability of these patients, they are still not conclusive. Repairing defects is considered only in patients with PVRi <4 WU/m2, is not indicated if 8 WU/7m2 is exceeded, and in patients with PVRi between 4 and 8 WU/m2 it is advised to perform a thorough assessment in expert reference centers. Other variables that help in making decisions are the type of shunt, age, PVR/SVR quotient, and Qp/Qs ratio. Some task groups, based on retrospective data, recommend the performance of AVT and/or occlusion test to collaborate on deciding about closure[1,66,67,68,69,70,75,76,77].
In adult patients with congenital heart diseases, it is advised to drop the use of PVRi and using absolute PVR, and proceed to close shunts with Qp/Qs >1.5 and PVR <5 WU, not advising closing pre-tricuspid shunts with PVR > 5 WU. The strategy to “treat and repair” is also proposed faced with performance of AVT to assess the shunt closure[62]. However, some groups still perform AVT and temporary occlusion test[77,78,79,80,81,82].
AVT in patients with PAH and congenital shunts differ from that made in idiopathic PAH. It is considered positive if there is a reduction in PVRi <4 WU/m2 and a proportion between PVR and SVR <0.33[81].
In the cases with ostium secundum or ductus atrial septal defect, a temporary occlusion of the defect (occlusion test) will exclude pulmonary hyperflow and may help in making decisions. Occlusion test will be considered positive if there is reduction in mPAP ≥25% and a reduction in the proportion between dPAP and diastolic systemic blood pressure (dSBP) of more than 50%[82].
It is important to emphasize that these recommendations and cutoff points are predominantly based on the opinion of experts, that there are no prospective studies that have identified reliable values that would predict pulmonary vascular disease reversion and normalization of hemodynamics after cardiac correction. The retrospective studies available are limited by selection biases, preoperative characteristics or incomplete follow-up.
7.3 Pulmonary arterial hypertension with small/matching congenital heart defect
The surgical or percutaneous repair in a late stage, when the patient has already developed pulmonary vascular disease, may transform unfavorably the prognosis, since it will significantly increase RV afterload, which will present progressive function impairment and finally right heart failure[83,84]. For this reason, it is important to make the decision to repair these defects in reference centers with experience on PAH associated to CHF, based not only on hemodynamic information, but also on all the clinical information of the patient.
8. PULMONARY ARTERIOGRAPHY IN CHRONIC THROMBOEMBOLIC PULMONARY HYPERTENSION
Traditional pulmonary arteriography is a fundamental tool in the diagnostic algorithm of CTEPH, being its final link and gold standard to confirm its presence[1,85]. On the other hand, one of the great advantages of this method is that it enables evaluating the anatomical feasibility both for surgical endarterectomy and pulmonary angioplasty with balloon[86,87].
It is an invasive test of pulmonary vascular anatomy, which could be made very safely, with low morbidity and mortality, in experienced centers and with properly trained staff.
After RHC, during the intervention proper, it is suggested to perform the pulmonary arteriography in patients with PH in whom there is suspicion of thromboembolic origin. It is necessary for this procedure to be made after hemodynamic measurements, since contrast injections may generate alterations in measurements.
To make an accurate assessment of the pulmonary tree, knowledge is required on pulmonary vasculature anatomy (Figure 5). The main pulmonary artery originates in the right ventricular infundibulum, anterior and at the left of the aorta. It follows a posteromedial direction until its bifurcation into the right and left pulmonary arteries. The right pulmonary artery follows an anterior course to the main right bronchus. It originates the right upper lobe branch within the mediastinum. The left pulmonary artery goes above the main left bronchus and descends posteriorly to the bronchus after originating the left upper lobe branch. The vessels then branch out, closely related to bronchial ramification within the lung.
For this test, venous access should be made, ideally guided by ultrasound, where a 6 French (Fr) introducer is used. Such access is usually femoral; however, in some specific cases, jugular and even brachial access could be used. For instance, faced with the presence of inferior vena cava filters, it is suggested to prevent femoral accesses. Through the 6 Fr introducer, a pigtail catheter is used (the most used catheter), guided by a 0.035 J wire toward the pulmonary artery and the right or left pulmonary artery is selectively cannulated. Once each of the pulmonary arteries is reached, we should apply three injections of contrast with the injector pump selectively. Each of the injections is made in different angiographic projections, posterior-anterior (PA, 0° right, 0° cranial), ipsilateral oblique (45° right for the right lung, 45° left for the left lung) and lateral (90° right for the right lung, 90° left for the left lung); for the lateral projection, it is important to raise both arms of the patient. It is necessary to do all projections because of the complexity of pulmonary anatomy and its arrangement in 3 dimensions (Figure 5). In the different angulations, it will be possible to better display the different branches in both lungs (Figure 6). To configure the injector pump, it should be loaded with enough contrast volume (120-180 ml) to do 6 injections. Ideally, low-osmolality and low-viscosity nonionic contrast (320 mgl/ml) is used; even in patients with petite body frames contrast could be diluted to 70%, adding 30% of saline solution. Each injection will have a total volume between 20 and 30 ml infused at a velocity of 20-25 ml/sec, taking into account a pressure limit between 600 and 800 PSI. It is important to take into account right ventricular function and the load conditions of the patient to determine the total volume of infusion and its velocity.
In some cases, digital subtraction angiography could be used. This technology allows to decrease the contrast dose and visualize pulmonary perfusion, especially in the subpleural region of the capillary phase, which is considered poor when it is less or equal to 1.5 cm in the lateral pleura in the posterior-anterior projection[88]. This finding is suggestive of the presence of small vessel disease and/or diffuse thrombosis. However, classifying the type of vascular lesion found in large vessels is more difficult.
It is important, when performing the arteriography, to take the acquisitions in apnea with maximum inspiration and warn patients about the possible adverse effects they may experience, such as intense heat for a few seconds or cough.
The classical angiographic findings in patients with PAH include normal or dilated central arteries with tapering or narrowing of more distal non-elastic small arteries. Pulmonary vessels tapering may also be observed in chronic thromboembolic disease; but in this scenario, tapering is regional and more proximal.
In the diagnosis of CTEPH, five types of lesions, classically associated to this pathology, are described. Among them, ring-like lesions (fibrotic retractions of the artery), webs (fibrous webs within the vessel), subocclusive lesions (arterial narrowing that only allows a minimum passage of contrast), total occlusions (complete interruption of the vessel) and tortuous lesions (severe tortuosity of the vessel)[89] (Figure 7). In this regard, it is important to highlight that pulmonary angioplasty is not feasible in all lesions. The prognosis and rate of complications depend on the type of lesion, so the rate of success is greater and the rate of complications is less in ring-like and web lesions. Total occlusions have a lower rate of success; and tortuous lesions a greater rate of complications[89].
The aim of angioplasty is to break and move intravascular membranes and septa to thus improve the passage of blood and distal perfusion. An improvement is observed immediately after balloon inflation, at the level of membranes or stenosis, in the degree of perfusion of the treated vessel, as well as increase in the contrast in pulmonary segments distal to the treated lesions, and an improvement in venous return toward the left atrium. These data state that angioplasty has been efficient[89,90].
Selective pulmonary arteriography allows to visualize with more detail, the type and location of the lesions, which is made with manual 4-8 ml injections of contrast through therapeutic catheters, usually during pulmonary angioplasty with balloon[89]. On the other hand, quantitative coronary angiography (QCA) may also be used, which uses the catheter diameter as reference and allows the estimation of vessel diameters, minimum luminal diameter, percentage of stenosis and length of obstruction.
During the invasive evaluation of these lesions, other methods could be used such as optical coherence tomography (OCT), intravascular ultrasound (IVUS) or the direct measurement of translesional pressure gradients (fractional flow reserve - FFR); however, none of these technologies are strongly recommended, or are used widely.
It is extremely important to discuss all pulmonary angiography findings along with an interdisciplinary group of professionals with experience in the diagnosis and treatment of patients with chronic thromboembolic pulmonary hypertension.
BIBLIOGRAPHY
- Galiè N, Humbert M, Vachiery J-L, et al. 2015 ESC/ERS Guidelines for the diagnosis and treatment of pulmonary hypertension. European Heart Journal. 2016;37:67-119.
- Simonneau G, Montani D, Celermajer DS, et al. Haemodynamic definitions and updated clinical classification of pulmonary hypertension. European Respiratory Journal. 2019;53:1801913.
- Geneva 15-17 October 1973. Primary Pulmonary Hypertension. Report on a WHO meeting. . http://www.wsphassociation.org/.
- Kovacs G, Berghold A, Scheidl S, Olschewski H. Pulmonary arterial pressure during rest and exercise in healthy subjects: a systematic review. European Respiratory Journal. 2009;34:888-894..
- Barst RJ, McGoon M, Torbicki A, et al. Diagnosis and differential assessment of pulmonary arterial hypertension. J Am Coll Cardiol. 2004;43:S40-S47.
- Naeije R, Vanderpool R, Dhakal BP, et al. Exercise-induced Pulmonary Hypertension. American Journal of Respiratory and Critical Care Medicine. 2013;187:576-583.
- Mazzei JA, Cáneva JO, Bosio MF, et al. Guías Argentinas de Consenso en Diagnóstico y Tratamiento de la Hipertensión Pulmonar. Revista Argentina de Cardiología. 2017;85.
- Hoeper MM, Lee SH, Voswinckel R, et al. Complications of Right Heart Catheterization Procedures in Patients With Pulmonary Hypertension in Experienced Centers. J Am Coll Cardiol. 2006;48:2546-2552.
- D’Alto M, Dimopoulos K, Coghlan JG, Kovacs G, Rosenkranz S, Naeije R. Right Heart Catheterization for the Diagnosis of Pulmonary Hypertension. Heart Failure Clinics. 2018;14:467-477.
- Vachiéry J-L, Tedford RJ, Rosenkranz S, et al. Pulmonary hypertension due to left heart disease. European Respiratory Journal. 2019;53:1801897.
- Kovacs G, Avian A, Pienn M, Naeije R, Olschewski H. READING PULMONARY VASCULAR PRESSURE TRACINGS How to handle the problems of zero leveling and respiratory swings. American Journal of Respiratory and Critical Care Medicine. Published online May 28, 2014:140528122914004.
- Rosenkranz S, Gibbs JSR, Wachter R, de Marco T, Vonk-Noordegraaf A, Vachiéry J-L. Left ventricular heart failure and pulmonary hypertension. European Heart Journal. 2016;37:942-954.
- HOEPER MM, MAIER R, TONGERS J, et al. Determination of Cardiac Output by the Fick Method, Thermodilution, and Acetylene Rebreathing in Pulmonary Hypertension. American Journal of Respiratory and Critical Care Medicine. 1999;160:535-541..
- Ryan JJ, Rich JD, Thiruvoipati T, Swamy R, Kim GH, Rich S. Current practice for determining pulmonary capillary wedge pressure predisposes to serious errors in the classification of patients with pulmonary hypertension. American Heart Journal. 2012;163:589-594.
- Deaño RC, Glassner-Kolmin C, Rubenfire M, et al. Referral of Patients With Pulmonary Hypertension Diagnoses to Tertiary Pulmonary Hypertension Centers. JAMA Internal Medicine. 2013;173:887.
- Montani D, Savale L, Natali D, et al. Long-term response to calcium-channel blockers in non-idiopathic pulmonary arterial hypertension. European Heart Journal. 2010;31:1898-1907.
- Galiè N, Channick RN, Frantz RP, et al. Risk stratification and medical therapy of pulmonary arterial hypertension. European Respiratory Journal. 2019;53:1801889.
- Mayeux JD, Pan IZ, Dechand J, et al. Management of Pulmonary Arterial Hypertension. Current Cardiovascular Risk Reports. 2021;15:2.
- Kanwar MK, Gomberg-Maitland M, Hoeper M, et al. Risk stratification in pulmonary arterial hypertension using Bayesian analysis. European Respiratory Journal. 2020;56:2000008.
- Cendón AA. El estudio hemodinámico: indicaciones del cateterismo derecho e izquierdo en el diagnóstico y seguimiento de la hipertensión pulmonar. Archivos de Bronconeumología. 2011;47:12-14.
- Amsallem M, Bagherzadeh SP, Boulate D, et al. Hemodynamic trajectories and outcomes in patients with pulmonary arterial hypertension. Pulmonary Circulation. 2020;10:1-12.
- Weatherald J, Boucly A, Chemla D, et al. Prognostic Value of Follow-Up Hemodynamic Variables After Initial Management in Pulmonary Arterial Hypertension. Circulation. 2018;137:693-704.
- Boucly A, Weatherald J, Savale L, et al. Risk assessment, prognosis and guideline implementation in pulmonary arterial hypertension. European Respiratory Journal. 2017;50:1700889.
- Kooranifar S, Naghshin R, Sezavar SH, Hajsadeghi S, Talebzadeh SM. Diagnostic value of chest spiral CT scan and Doppler echocardiography compared to right heart catheterization to predict pulmonary arterial hypertension in patients with scleroderma. Acta Biomed. 2021;92:e2021074.
- Benza RL, Miller DP, Gomberg-Maitland M, et al. Predicting Survival in Pulmonary Arterial Hypertension. Circulation. 2010;122:164-172.
- Rosenkranz S, Preston IR. Right heart catheterisation: best practice and pitfalls in pulmonary hypertension. European Respiratory Review. 2015;24:642-652.
- Krishnan A, Markham R, Savage M, Wong Y-W, Walters D. Right Heart Catheterisation: How To Do It. Heart, Lung and Circulation. 2019;28:e71-e78.
- Lauga LA, Lauga A, D’ortencio A. A Lauga y A D’Ortencio Catéter de Swan-Ganz-Parte I Monitoreo de las presiones de la arteria pulmonar Catéter de Swan-Ganz Parte I GUIA DE MONITOREO HEMODINAMICO. Insuf Cardiac. 2007;2.
- Lauga A, D’Ortencio A. Monitoreo de las presiones de la arteria pulmonar. Catéter de Swan-Ganz. Parte II. Insuficiencia cardíaca . 2007;2:48-54.
- Lauga A, Perrone S v. Monitoreo de las presiones de la arteria pulmonar Catéter de Swan-Ganz Parte III. Rev Insuf Cardíaca. 2007;2.
- Lauga A, Perel C, Perrone SV. Monitoreo de las presiones de la arteria pulmonar: catéter de Swan-Ganz. Parte IV. Insuficiencia Cardíaca. 2007;2:143-148.
- Montrief T, Auerbach J, Cabrera J, Long B. Use of Point-of-Care Ultrasound to Confirm Central Venous Catheter Placement and Evaluate for Postprocedural Complications. The Journal of Emergency Medicine. 2021;60:637-640.
- Saugel B, Scheeren TWL, Teboul J-L. Ultrasound-guided central venous catheter placement: a structured review and recommendations for clinical practice. Critical Care. 2017;21:225.
- Chen Y, Shlofmitz E, Khalid N, et al. Right Heart Catheterization-Related Complications. Cardiology in Review. 2020;28:36-41.
- Kovacs G, Avian A, Olschewski A, Olschewski H. Zero reference level for right heart catheterisation. European Respiratory Journal. 2013;42:1586-1594.
- Winsor T, Burch GE. Phlebostatic Axis and Phlebostatic Level, Reference Levels for Venous Pressure Measurements in Man. Experimental Biology and Medicine. 1945;58:165-169.
- Kelly CR, Rabbani LE. Pulmonary-Artery Catheterization. New England Journal of Medicine. 2013;369:e35.
- Hoeper MM, Bogaard HJ, Condliffe R, et al. Definitions and Diagnosis of Pulmonary Hypertension. J Am Coll Cardiol. 2013;62:D42-D50.
- Duarte M, Dvorkin MA, Lentini N. Función sistólica y diastólica. Gasto cardíaco. In: Cardinali DP, Dvorkin MA, Iermoli R, eds. Best &Taylor. Bases Fisiológicas de La Práctica Médica. 14th ed. Editorial Medica Panamericana; 2010:265-284.
- Lankhaar J-W, Westerhof N, Faes TJC, et al. Pulmonary vascular resistance and compliance stay inversely related during treatment of pulmonary hypertension. European Heart Journal. 2008;29:1688-1695.
- Mahapatra S, Nishimura RA, Sorajja P, Cha S, McGoon MD. Relationship of Pulmonary Arterial Capacitance and Mortality in Idiopathic Pulmonary Arterial Hypertension. J Am Coll Cardiol. 2006;47:799-803.
- Lim Y, Low T, Chan SP, et al. Does pulmonary artery pulsatility index predict mortality in pulmonary arterial hypertension? ESC Heart Failure. 2021;8:3835-3844.
- Palazzini M, Dardi F, Manes A, et al. Pulmonary hypertension due to left heart disease: analysis of survival according to the haemodynamic classification of the 2015 ESC/ERS guidelines and insights for future changes. European Journal of Heart Failure. 2018;20:248-255.
- Barańska-Pawełczak K, Wojciechowska C, Opara M, Jacheć W. Nitric Oxide Stroke Volume Index as a New Hemodynamic Prognostic Parameter for Patients with Pulmonary Arterial Hypertension. Journal of Clinical Medicine. 2020;9:2939.
- Ghio S, Schirinzi S, Pica S. Pulmonary arterial compliance: How and why should we measure it? Global Cardiology Science and Practice. 2015;2015:58.
- Mayfield JJ, Papolos A, Vasti E, de Marco T, Tison GH. Pulmonary arterial capacitance predicts outcomes in patients with pulmonary hypertension independent of race/ethnicity, sex, and etiology. Respiratory Medicine. 2020;163:105891.
- Benza RL, Gomberg-Maitland M, Elliott CG, et al. Predicting Survival in Patients With Pulmonary Arterial Hypertension. Chest. 2019;156:323-337.
- Rich S, Brundage BH. High-dose calcium channel-blocking therapy for primary pulmonary hypertension: evidence for long-term reduction in pulmonary arterial pressure and regression of right ventricular hypertrophy. Circulation. 1987;76:135-141.
- Rich S, Kaufmann E, Levy PS. The Effect of High Doses of Calcium-Channel Blockers on Survival in Primary Pulmonary Hypertension. New England Journal of Medicine. 1992;327:76-81.
- Sitbon O, Humbert M, Jaïs X, et al. Long-Term Response to Calcium Channel Blockers in Idiopathic Pulmonary Arterial Hypertension. Circulation. 2005;111:3105-3111.
- Langleben D, Orfanos S. Vasodilator responsiveness in idiopathic pulmonary arterial hypertension: identifying a distinct phenotype with distinct physiology and distinct prognosis. Pulmonary Circulation. 2017;7:588-597.
- Hemnes AR, Trammell AW, Archer SL, et al. Peripheral Blood Signature of Vasodilator-Responsive Pulmonary Arterial Hypertension. Circulation. 2015;131:401-409.
- Hemnes AR, Zhao M, West J, et al. Critical Genomic Networks and Vasoreactive Variants in Idiopathic Pulmonary Arterial Hypertension. American Journal of Respiratory and Critical Care Medicine. 2016;194:464-475.
- Medarov BI, Judson MA. The role of calcium channel blockers for the treatment of pulmonary arterial hypertension: How much do we actually know and how could they be positioned today? Respiratory Medicine. 2015;109:557-564.
- Kovacs G, Berghold A, Scheidl S, Olschewski H. Pulmonary arterial pressure during rest and exercise in healthy subjects: a systematic review. European Respiratory Journal. 2009;34:888-894.
- Esfandiari S, Wolsk E, Granton D, et al. Pulmonary Arterial Wedge Pressure at Rest and During Exercise in Healthy Adults: A Systematic Review and Meta-analysis. Journal of Cardiac Failure. 2019;25:114-122.
- Robbins IM, Hemnes AR, Pugh ME, et al. High Prevalence of Occult Pulmonary Venous Hypertension Revealed by Fluid Challenge in Pulmonary Hypertension. Circulation: Heart Failure. 2014;7:116-122.
- Fujimoto N, Borlaug BA, Lewis GD, et al. Hemodynamic Responses to Rapid Saline Loading. Circulation. 2013;127:55-62.
- Andersen MJ, Ersbøll M, Bro-Jeppesen J, et al. Exercise Hemodynamics in Patients With and Without Diastolic Dysfunction and Preserved Ejection Fraction After Myocardial Infarction. Circulation: Heart Failure. 2012;5:444-451.
- Andersen MJ, Olson TP, Melenovsky V, Kane GC, Borlaug BA. Differential Hemodynamic Effects of Exercise and Volume Expansion in People With and Without Heart Failure. Circulation: Heart Failure. 2015;8:41-48.
- Borlaug BA. Invasive Assessment of Pulmonary Hypertension. Circulation: Heart Failure. 2014;7:2-4.
- Baumgartner H, de Backer J, Babu-Narayan S v, et al. 2020 ESC Guidelines for the management of adult congenital heart disease. European Heart Journal. 2021;42:563-645.
- Simonneau G, Galiè N, Rubin LJ, et al. Clinical classification of pulmonary hypertension. J Am Coll Cardiol. 2004;43:S5-S12.
- Duffels MGJ, Engelfriet PM, Berger RMF, et al. Pulmonary arterial hypertension in congenital heart disease: An epidemiologic perspective from a Dutch registry. International Journal of Cardiology. 2007;120:198-204.
- Vogel M, Berger F, Kramer A, Alexi-Meshkishvili V, Lange PE. Incidence of secondary pulmonary hypertension in adults with atrial septal or sinus venosus defects. Heart. 1999;82:30-33.
- Opotowsky AR. Clinical Evaluation and Management of Pulmonary Hypertension in the Adult With Congenital Heart Disease. Circulation. 2015;131:200-210.
- Gatzoulis MA, Beghetti M, Landzberg MJ, Galiè N. Pulmonary arterial hypertension associated with congenital heart disease: Recent advances and future directions. International Journal of Cardiology. 2014;177:340-347.
- Alkhodair A, Tsang MYC, Cairns JA, et al. Comparison of thermodilution and indirect Fick cardiac outputs in pulmonary hypertension. International Journal of Cardiology. 2018;258:228-231.
- Lopes AA, O’Leary PW. Measurement, interpretation and use of haemodynamic parameters in pulmonary hypertension associated with congenital cardiac disease. Cardiology in the Young. 2009;19:431-435.
- Lopes AA, O’Leary PW. Measurement, interpretation and use of hemodynamic parameters. Cardiology in the Young. 2009;19:8-12.
- Tamimi O, Mohammed MHA. Pulmonary Vascular Resistance Measurement Remains Keystone in Congenital Heart Disease Management. Frontiers in Cardiovascular Medicine. 2021;8.
- Berger R. Possibilities and impossibilities in the evaluation of pulmonary vascular disease in congenital heart defects. European Heart Journal. 2000;21:17-27.
- Wood P. The Eisenmenger Syndrome: I. BMJ. 1958;2:701-709.
- Wood P. The Eisenmenger Syndrome: II. BMJ. 1958;2:755-762.
- Moller JH, Patton C, Varco RL, Lillehei CW. Late results (30 to 35 years) after operative closure of isolated ventricular septal defect from 1954 to 1960. The American Journal of Cardiology. 1991;68:1491-1497.
- Viswanathan S, Kumar RK. Assessment of operability of congenital cardiac shunts with increased pulmonary vascular resistance. Catheterization and Cardiovascular Interventions. 2008;71:665-670.
- Lopes AA, Barst RJ, Haworth SG, et al. Repair of Congenital Heart Disease with Associated Pulmonary Hypertension in Children: What are the Minimal Investigative Procedures? Consensus Statement from the Congenital Heart Disease and Pediatric Task Forces, Pulmonary Vascular Research Institute (PVRI). Pulmonary Circulation. 2014;4:330-341.
- Dimopoulos K, Wort SJ, Gatzoulis MA. Pulmonary hypertension related to congenital heart disease: a call for action. European Heart Journal. 2014;35:691-700.
- Brida M, Gatzoulis MA. Pulmonary arterial hypertension in adult congenital heart disease. Heart. 2018;104:1568-1574.
- Balzer DT, Kort HW, Day RW, et al. Inhaled Nitric Oxide as a Preoperative Test (INOP Test I): the INOP Test Study Group. Circulation. 2002;106:I76-81.
- Beghetti M, Galiè N, Bonnet D. Can “Inoperable” Congenital Heart Defects Become Operable in Patients with Pulmonary Arterial Hypertension? Dream or Reality? Congenital Heart Disease. 2012;7:3-11.
- Sánchez-Recalde Á, Oliver JM, Galeote G, et al. Atrial Septal Defect With Severe Pulmonary Hypertension in Elderly Patients: Usefulness of Transient Balloon Occlusion. Revista Española de Cardiología (English Edition). 2010;63:860-864.
- Galie N, Manes A, Palazzini M, et al. Management of Pulmonary Arterial Hypertension Associated with Congenital Systemic-to-Pulmonary Shunts and Eisenmenger???s Syndrome. Drugs. 2008;68:1049-1066.
- Manes A, Palazzini M, Leci E, Bacchi Reggiani ML, Branzi A, Galie N. Current era survival of patients with pulmonary arterial hypertension associated with congenital heart disease: a comparison between clinical subgroups. European Heart Journal. 2014;35:716-724.
- Wilkens H, Konstantinides S, Lang IM, et al. Chronic thromboembolic pulmonary hypertension (CTEPH): Updated Recommendations from the Cologne Consensus Conference 2018. International Journal of Cardiology. 2018;272:69-78.
- Kim NH, Delcroix M, Jais X, et al. Chronic thromboembolic pulmonary hypertension. European Respiratory Journal. 2019;53:1801915.
- Feinstein JA, Goldhaber SZ, Lock JE, Ferndandes SM, Landzberg MJ. Balloon Pulmonary Angioplasty for Treatment of Chronic Thromboembolic Pulmonary Hypertension. Circulation. 2001;103:10-13.
- Remy-Jardin M, Ryerson CJ, Schiebler ML, et al. Imaging of pulmonary hypertension in adults: a position paper from the Fleischner Society. European Respiratory Journal. 2021;57:2004455.
- Kawakami T, Ogawa A, Miyaji K, et al. Novel Angiographic Classification of Each Vascular Lesion in Chronic Thromboembolic Pulmonary Hypertension Based on Selective Angiogram and Results of Balloon Pulmonary Angioplasty. Circulation: Cardiovascular Interventions. 2016;9.
- Shimokawahara H, Ogawa A, Matsubara H. Balloon pulmonary angioplasty for chronic thromboembolic pulmonary hypertension: advances in patient and lesion selection. Current Opinion in Pulmonary Medicine. 2021;27:303-310.